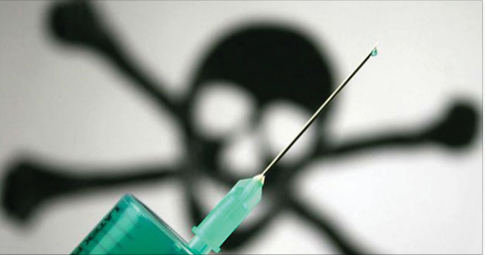
Immunization Safety Review: SV40 Contamination of Polio Vaccine and Cancer
A Report on SV40 Vaccine Contamination by the Institute of Medicine (US) Immunization Safety Review Committee; Stratton K, Almario DA, McCormick MC, editors.
Immunization to protect children and adults from many infectious diseases is one of the greatest achievements of public health. Immunization is not without risks, however. It is well established, for example, that some influenza vaccines have been associated with a risk of Guillain-Barré syndrome and that vaccines sometimes produce anaphylactic shock. Given the widespread use of vaccines, state mandates requiring vaccination of children for entry into school, college, or day care, and the importance of ensuring that trust in immunization programs is justified, it is essential that safety concerns receive assiduous attention.
The Immunization Safety Review Committee was established by the Institute of Medicine (IOM) to evaluate the evidence on possible causal associations between immunizations and certain adverse outcomes, and to then present conclusions and recommendations. The committee’s mandate also includes assessing the broader significance for society of these immunization safety issues.
In this fifth report in a series, the committee examines the hypothesis that exposure to polio vaccine contaminated with simian virus 40 (SV40) can cause certain types of cancer.
THE CHARGE TO THE COMMITTEE
Challenges to the safety of immunizations are prominent in public and scientific debate. Given these persistent and growing concerns about immunization safety, the Centers for Disease Control and Prevention (CDC) and the National Institutes of Health (NIH) recognized the need for an independent, expert group to address immunization safety in a timely and objective manner. The IOM has been involved in such issues since the 1970s. (A brief chronology can be found in Appendix C.) In 1999, as a result of IOM’s previous work and its access to independent scientific experts, CDC and NIH began a year of discussions with IOM to develop the Immunization Safety Review project, which addressed both emerging and existing vaccine safety issues.
The Immunization Safety Review Committee is responsible for examining a broad variety of immunization safety concerns. Committee members have expertise in pediatrics, neurology, immunology, internal medicine, infectious diseases, genetics, epidemiology, biostatistics, risk perception and communication, decision analysis, public health, nursing, and ethics. While all the committee members share the view that immunization is generally beneficial, none of them has a vested interest in the specific immunization safety issues that come before the group. Additional discussion of the committee composition can be found in the Foreword written by Dr. Harvey Fineberg, President of the IOM.
The committee is charged with examining three immunization safety hypotheses each year during the three-year study period (2001–2003). These hypotheses are selected by the Interagency Vaccine Group, whose members represent several units of the Department of Health and Human Services (DHHS)— the National Vaccine Program Office, the National Immunization Program, and the National Center for Infectious Diseases at the CDC, the National Institute for Allergy and Infectious Diseases at the NIH, the Food and Drug Administration (FDA), the National Vaccine Injury Compensation Program at the Health Resources and Services Administration (HRSA), and the Centers for Medicare and Medicaid Services (CMS, formerly the Health Care Financing Administration), as well as the Department of Defense and the Agency for International Development.
For each topic, the Immunization Safety Review Committee reviews relevant literature and submissions by interested parties, holds an open scientific meeting, and directly follows the open meeting with a 1- to 2-day closed meeting to formulate its conclusions and recommendations. The committee’s findings are released to the public in a brief consensus report 60 to 90 days after its meeting.
The committee is charged with assessing both the scientific evidence regarding the hypotheses under review and the significance of the issues for society.
-
The scientific assessment has two components: an examination of the epidemiologic and clinical evidence regarding a possible causal relationship between exposure to the vaccine and the adverse event, and an examination of theory and experimental evidence from human or animal studies regarding biological mechanisms that might be relevant to the hypothesis.
-
The significance assessment addresses such considerations as the burden of the health risks associated with the vaccine-preventable disease and with the adverse event. Other considerations may include the perceived intensity of public or professional concern, or the feasibility of additional research to help resolve scientific uncertainty regarding causal associations.
The findings of the scientific and significance assessments provide the basis for the committee’s recommendations regarding the public health response to the issue. In particular, the committee addresses needs for a review of immunization policy, for current and future research, and for effective communication strategies. See Figure 1 for a schematic representation of the committee’s charge.

Figure 1
Committee Charge
THE STUDY PROCESS
The committee held an initial organizational meeting in January 2001. CDC and NIH presented the committee’s charge at the meeting, and the committee conducted a general review of immunization safety concerns. At this initial meeting, the committee also determined the basic methodology to be used for assessing causality for the hypotheses to be considered at subsequent meetings. A website (www.iom.edu/imsafety) and a listserv were created to provide public access to information about the committee’s work and to facilitate communication with the committee. The conclusions and recommendations of the committee’s previous reports (see Box 1) are summarized in Appendix A.

BOX 1
Previous Reports by the Immunization Safety Review Committee. Immunization Safety Review: Measles-Mumps-Rubella Vaccine and Autism (2001a)
For its evaluation of the hypothesis on SV40-contaminated polio vaccine and cancer, the committee held an open scientific meeting in July 2002 (see Appendix B) to hear presentations on issues germane to the topic. The presentations to the committee at the open meeting are available in electronic form (audio files and slides) on the project website (www.iom.edu/imsafety). In addition, the committee reviewed an extensive collection of material, primarily from the published, peer-reviewed scientific and medical literature. A list of the materials reviewed by the committee, including many items not cited in this report, can be found on the project’s website.
THE FRAMEWORK FOR SCIENTIFIC ASSESSMENT
Causality
The Immunization Safety Review Committee has adopted the framework for assessing causality developed by previous IOM committees (IOM, 1991, 1994), convened under the congressional mandate of P.L. 99–660 to address questions of immunization safety. The categories of causal conclusions used by the committee are as follows:
- 1.
-
No evidence
- 2.
-
Evidence is inadequate to accept or reject a causal relationship
- 3.
-
Evidence favors rejection of a causal relationship
- 4.
-
Evidence favors acceptance of a causal relationship
- 5.
-
Evidence establishes a causal relationship.
Assessments begin from a position of neutrality regarding the specific vaccine safety hypothesis under review. That is, there is no presumption that a specific vaccine (or vaccine component) does or does not cause the adverse event in question. The weight of the available clinical and epidemiologic evidence determines whether it is possible to shift from that neutral position to a finding for causality (“the evidence favors acceptance of a causal relationship”) or against causality (“the evidence favors rejection of a causal relationship”). The committee does not conclude that the vaccine does not cause the adverse event merely if the evidence is inadequate to support causality. Instead, it maintains a neutral position, concluding that the “evidence is inadequate to accept or reject a causal relationship.”
Although no firm rules establish the amount of evidence or the quality of the evidence required to support a specific category of causality conclusion, the committee uses standard epidemiologic criteria to guide its decisions. The most definitive category is “establishes causality,” which is reserved for those relationships where the causal link is unequivocal, as with the oral polio vaccine and vaccine-associated paralytic polio or with anaphylactic reactions to vaccine administration. The next category, “favors acceptance” of a causal relationship, reflects evidence that is strong and generally convincing, although not firm enough to be described as unequivocal or established. “Favors rejection” is the strongest category in the negative direction. The category of “establishes no causal relationship” is not used because it is virtually impossible to prove the absence of a relationship with the same surety that is possible in establishing its presence.
If the evidence is not reasonably convincing either in support of or against causality, the category “inadequate to accept or reject a causal relationship” is used. Evidence that is sparse, conflicting, of weak quality, or merely suggestive either toward or away from causality falls into this category. Some authors of similar assessments use phrases such as “the evidence does not presently support a causal association.” The committee believes, however, that such language does not make the important distinction between evidence indicating that a relationship does not exist (category 3) and evidence that is indeterminate with regard to causality (category 2). The category of “no evidence” is reserved for those cases in which there is a complete absence of clinical or epidemiologic evidence.
The sources of evidence considered by the committee in its scientific assessment of causality include epidemiologic and clinical studies directly addressing the question at hand. That is, the data are specifically related to the effects of the vaccine(s) under review and the adverse health outcome(s) under review—in the case of this report, the effects of SV40 contamination of the polio vaccine and the risk for certain types of cancer.
Epidemiologic studies carry the most weight in a causality assessment. These studies measure health-related exposures and outcomes in a defined set of subjects and make inferences about the nature and strength of associations between exposures and outcomes in the overall population from which the study sample was drawn. Epidemiologic studies can be categorized as observational or experimental (clinical trial), and as uncontrolled (descriptive) or controlled (analytic). Among these various study designs, experimental studies generally have the advantage of random assignment to exposures and are therefore the most influential in assessing causality. Uncontrolled observational studies are important but are generally considered less definitive than controlled studies. In uncontrolled observational studies, where observations are made over time, confounding from factors such as changing case definitions or improving case detection may affect the apparent incidence and prevalence of the adverse outcomes studied.
By themselves, case reports and case series are generally inadequate to establish causality. Despite the limitations of case reports, the causality argument for at least one vaccine-related adverse event (the relationship between vaccines containing tetanus toxoid and Guillain-Barré syndrome) was strengthened most by a single, well-documented case report on recurrence of the adverse event following re-administration of the vaccine, a situation referred to as a “rechallenge” (IOM, 1994).
Biological Mechanisms
Evidence considered in the scientific assessment of biological mechanisms1 includes human, animal, and in vitro studies related to biological or pathophysiological processes by which immunizations could cause an adverse event. When other evidence of causality is available, biological data add supportive evidence, but they cannot prove causality on their own.
This committee is often faced with a set of circumstances in which the epidemiologic evidence is judged inadequate to accept or reject a causal association between a vaccine exposure and an adverse event of concern. It is then left with the task of examining proposed or conceivable biological mechanisms that might be operating if an epidemiologically sound association could be shown between a vaccine exposure and an adverse event.
In any case, the committee’s causality assessments must be guided by the current understanding of biological processes. In fact, the current thinking on a possible biological explanation for a relationship between immunization and an adverse event will influence the design of a good epidemiologic analysis. The essential consideration of “confounders” in epidemiologic studies depends on an understanding of the biological phenomena that could underlie or explain the observed statistical relationship. A statistical observation can be considered as evidence of causality only when important confounders are considered. However, without evidence of a statistical association or convincing clinical evidence, biological mechanisms cannot be invoked as proof of causality.
The identification of sound biological mechanisms can also influence the development of an appropriate research agenda and give support for policymakers, who frequently must make decisions without having complete information regarding causality. In addition, there is often value in investigating and understanding possible biological mechanisms even if the available epidemiologic evidence suggests the absence of a causal association. A review of biological data could give support to the negative causality assessment, for example, or it could prompt a reconsideration or further investigation of the epidemiologic findings. If new epidemiologic studies were to question the existing causality assessment, the biological data could gain prominence in the new assessments.
The committee has established three general categories of evidence on biological mechanisms:
- 1.
-
Theory only. A reasonable mechanism can be hypothesized that is commensurate with scientific knowledge and that does not contradict known physical and biological principles, but has not been demonstrated in whole or in part in humans or in animal models. Postulated mechanisms by which a vaccine might cause a specific adverse event but for which no coherent theory exists would not meet the criteria for this category. Thus, “theoretical only” is not a default category, but one that requires thoughtful and biologically meaningful suppositions.
- 2.
-
Experimental evidence that the mechanism operates in animals, in vitro systems, or humans. Experimental evidence often describes effects on just one or a few of the steps in the pathological process required for expression of disease. Showing that multiple components of the theoretical pathways operate in reasonable experimental models increases confidence that the mechanisms could possibly result in disease in humans. The evidence can be derived under highly contrived conditions. For example, achieving the results of interest may require extensive manipulation of the genetics of an animal system, or in vivo or in vitro exposures to vaccine antigen that are extreme in terms of dose, route, or duration. Other experimental evidence is derived under less contrived conditions. For example, a compelling animal or in vitro model exists whereby administration of a vaccine antigen under conditions similar to human use results in a pathologic process analogous to a human disease pathology. Mechanistic evidence also could come from studies in humans, but this is distinct from the evidence (about incidence of adverse events following immunization) that derives from randomized controlled trials or other population-based epidemiologic studies, which contribute to the causality assessment.
- 3.
-
Evidence that the mechanism results in known disease in humans. For example, the wild-type infection causes the adverse health outcome, or another vaccine has been demonstrated to cause the same adverse outcome by the same or a similar mechanism. Data from population-based studies of the effects of the vaccine administration on the occurrence of the adverse outcomes under review are not considered evidence regarding the biological mechanisms but rather as evidence regarding causality.
If the committee identifies evidence of biological mechanisms that could be operational, it will offer a summary judgment of that body of evidence as weak, moderate, or strong. Although the committee tends to judge biological evidence in humans as “stronger” than biological evidence from highly contrived animal models or in vitro systems, the summary judgment of the strength of the evidence also depends on both the quantity (e.g., number of studies or number of subjects in a study) and quality (e.g., the nature of the experimental system or study design) of the evidence. Obviously, the conclusions drawn from this review depend on both the specific data and on scientific judgment. To ensure that its own summary judgment is defensible, the committee intends to be as explicit as possible regarding the strengths and limitations of the biological data.
Published and Unpublished Data
Published reports carry the most weight in the committee’s assessment because their methods and findings are laid out in enough detail to be assessed. Furthermore, published works, which undergo a rigorous peer review, are subject to comment and criticism by the entire scientific community. In general, the committee cannot rely heavily on unpublished data in making its scientific assessments (regarding either causality or biological mechanisms) because they usually lack comment and criticism and must therefore be interpreted with caution. The committee also relies on editorial and peer review procedures to ensure the disclosure of potential conflicts of interest that might be related to the source of funding for the research study. Immunization safety studies and other data reviewed by the committee are funded by a variety of sources, including—NIH, CDC, vaccine manufacturers, research advocacy organizations, or foundations. The committee does not investigate the source of funding of the published research reports it reviews, nor does the funding source influence the committee’s interpretation of the evidence.
Unpublished data and other reports that have not undergone peer review do have value, however, and are often considered by the committee. They might be used, for example, in support of a body of published, peer-reviewed literature with similar findings. If the committee concluded that the unpublished data were well described, had been obtained using sound methodology, and presented very clear results, the committee could report, with sufficient caveats in the discussion, how the unpublished data fit with the entire body of published literature. Only in extraordinary circumstances, however, could an unpublished study refute a body of published literature.
The Immunization Safety Review Committee’s scope of work includes consideration of clinical topics for which high-quality experimental studies are rarely available. Many other panels making clinical recommendations using evidence-based methods are able to require that randomized trials be available to reach strong conclusions. However, the IOM Committee was convened specifically to assess topics that are of immediate concern and for which data of any kind may just be emerging. Thus, given the unique nature of this project, the committee decided that it was important to review and consider as much information as possible, including unpublished information. The committee does not perform primary or secondary analyses of unpublished data, however. In reviewing unpublished material, the committee applies generally accepted standards for assessing the quality of scientific evidence, as described above. (All unpublished data reviewed by the committee and cited in this report are available—in the form reviewed by the committee—through the public access files of the National Academies. Information about the public access files is available at 202– 334–3543 or www.national-academies.org/publicaccess.)
UNDER REVIEW: SV40 CONTAMINATION OF POLIO VACCINE AND CANCER
Polio vaccines were developed to prevent poliomyelitis, a highly contagious viral disease that was once common worldwide (See Box 2 for the chronology of polio vaccines used in the United States). Most infections are asymptomatic, but the most widely recognized form of polio is an infection of the central nervous system that results in paralysis of the limbs or respiratory muscles. During the first half of the 20th century, polio was at epidemic levels in the United States, peaking at more than 20,000 reported cases of paralytic disease in 1952 (CDC, 2000). Following the introduction of a vaccine against polio in 19552, the incidence of the disease rapidly declined. By 1965, only 61 paralytic cases were reported in the United States (CDC, 2002a). In 1994, the entire Western Hemisphere was declared free of indigenous wild poliovirus (CDC, 1994).

BOX 2
Chronology of Polio Vaccines Licensed in the United States. 1955 Inactivated polio vaccine (IPV) 1961 Live attenuated polio vaccine (OPV), Type 1
Despite its great value in controlling a devastating disease, polio vaccine is a source of concern because at least some of the vaccines used between 1955 and 1963, when more than 98 million persons were vaccinated in the United States, are known to have been contaminated with SV40. SV40 is a polyomavirus that commonly infects certain species of Asian macaques, especially the rhesus monkey. Other polyomaviruses, which are generally species-specific, include the BK and JC viruses of humans. Polyomaviruses are a genus of the papovavirus family of DNA viruses. This family also includes the papillomaviruses, of which one—human papillomavirus (HPV)—is causally associated with cervical cancer.
SV40 and other polyomaviruses generally produce inapparent infection in immunocompetent members of their natural host species. SV40 and the closely related human polyomaviruses BK and JC typically reside in renal epithelial cells. These viruses can, however, spread to other tissues and produce pathological effects in immunocompromised hosts or in non-host species. In fact, the presence of SV40 in polio vaccine produced from macaque kidney cell cultures was originally identified because of the cytopathological effects of the contaminated vaccine in African green monkey kidney cell cultures (Sweet and Hilleman, 1960). As another example, the JC virus is known to cause progressive multifocal leukoencephalopathy in immunocompromised humans.
With continuing controversy about the role of SV40 in human cancers (Brown and Lewis, 1998; Klein et al., 2002), the Interagency Vaccine Group asked the Immunization Safety Review Committee to address the question of whether exposure to the SV40-contaminated polio vaccine causes cancer in humans. The very abundance of new and emerging literature on the oncogenic potential of SV40 and its association with certain cancers suggests that it is not too late to try to resolve the question of whether SV40 contamination of polio vaccines could cause cancers in humans. Four forms of human cancer— mesothelioma, osteosarcoma, ependymoma, and non-Hodgkin’s lymphoma (NHL)—have been linked to SV40 in animal studies.
Polio Vaccines and SV40
Efforts to develop a vaccine effective against the three distinct types of the poliovirus began in the 1930s, but progress was hindered by the difficulty of producing an adequate supply of virus in the laboratory. The development in 1949 of a technique for growing the virus in tissue cultures (Enders et al., 1949) was soon followed by successful trials of a trivalent killed-virus vaccine. In 1954, 400,000 of 1.8 million children from the United States, Canada, and Finland actually received the potentially contaminated inactivated poliovirus vaccine (IPV) developed by Salk and colleagues (Francis et al., 1957). The vaccine was licensed for use in the United States in 1955. Vaccines that used a live attenuated virus and were administered orally were also developed, initially in a monovalent form. The trivalent oral poliovirus vaccine (OPV) known as the Sabin vaccine was licensed in the United States in 1963.
The tissue cultures used to grow poliovirus for these vaccines came from kidneys of rhesus and cynomolgus macaques.3 In 1960, Sweet and Hilleman (1960) reported that these tissues could be infected with SV40, a previously unknown virus that commonly infects rhesus macaques. Soon after its discovery, SV40 was shown to be able to produce tumors in hamsters and to transform human cells in culture (Eddy et al., 1961, 1962; Girardi et al., 1962; Koprowski et al., 1962; Shein and Enders, 1962a,b). Testing confirmed that some of the tissue cultures used in producing IPV and OPV were contaminated with SV40. In 1961, the U.S. government established requirements for testing to verify that all new lots of polio vaccine are free of SV40 (Egan, 2002). Potentially contaminated vaccine from previously approved lots of IPV was not recalled, however, and might have been used until early 1963.
IPV administered between 1955 and 1963 to about 98 million children and adults is assumed to be the primary source of human exposure to SV40 in the United States.4 In addition, experimental lots of OPV contaminated with SV40 was administered to about 10,000 people participating in clinical trials between 1959 and 1961. Recipients of the oral vaccine, in contrast to those receiving contaminated IPV, did not develop an antibody response to SV40 (as reviewed in Shah and Nathanson, 1976). This suggests that IPV, not OPV, resulted in the infection of humans with SV40. Nonetheless, concerns about the validity, and in particular the specificity for SV40, of the serologic testing create some uncertainty about this conclusion.
Details of the level and extent of the contamination of IPV are unavailable. Because the process used to inactivate the poliovirus was less effective against SV40, IPV could have included killed or live SV40. Furthermore, manufacturers used different types of cell cultures, and some were less vulnerable to contamination (Shah and Nathanson, 1976). Tests of stored samples of the vaccine that had been administered in the United States from May through July in 1955 found various levels of SV40 contamination, with some vaccine showing no contamination (Fraumeni et al., 1963). From these data, Shah and Nathanson (1976) estimated that 10% to 30% of IPV contained live SV40 and that similar percentages of the approximately 98 million Americans who had been vaccinated by 1961 were exposed to live SV40.
While it is certain that many people were directly exposed to SV40 through injections of IPV, two related matters remain unresolved. First, it is possible that some portion of the population might have been exposed to SV40 before IPV was introduced (Shah et al., 1972; Geissler et al., 1985). Second, it is unclear whether the SV40 received through the vaccine could be transmitted within the population once contaminated vaccine was no longer in use. A discussion of other possible sources of SV40 exposure is described in the section on biological mechanisms.
Cancers
In rodents, the injection of SV40 has been associated primarily with four types of cancer: mesothelioma, osteosarcoma, ependymoma, and lymphomas. Investigators have also detected SV40 DNA in human forms of these cancers. All but NHL are very rare, and they are described briefly here.
Mesothelioma
Malignant mesothelioma arises in the mesothelial cells that form a membrane around various internal organs. The most common form involves the pleura, which surround the lungs (Ho et al., 2001). The best-known disease risk factor in the United States and other industrialized countries is exposure to asbestos. Asbestos exposure does not account for all cases of malignant mesothelioma, however. Other suspected risk factors include radiation exposure and SV40. Genetic susceptibility may also play a role (Roushdy-Hammady et al., 2001).
Pleural mesothelioma was first described in 1931, and the incidence of the disease has increased steadily since then. In the United States, 2,000 to 3,000 new cases are diagnosed each year, which translates into a rate of 1.1/100,000 based on data from the Surveillance, Epidemiology, and End Results Program (SEER) for 19995 (Ho et al., 2001; Ries et al., 2002). Mesothelioma occurs predominately in men, with a 5:1 male-to-female ratio (Ho et al., 2001) and incidence is highest in men ages 75–84, with a rate of 16.2/100,000 (Ries et al., 2002). The marked difference is attributed to a greater occupational exposure to asbestos among men than women. The latency period for mesotheliomas linked to asbestos exposure can range from 30 to 50 years, but the disease can occur at any age (NIH, 2002).
Symptoms of pleural mesothelioma include shortness of breath, non-pleuritic chest pain, with evidence from physical or radiographic examination of unilateral or asymmetric bilateral pleural effusions. Symptoms worsen over time as the tumor grows. Treatment may include surgery, radiation, and chemotherapy. Median survival time following diagnosis generally ranges between 4 and 18 months (reviewed by Ho et al., 2001), with prognosis related to factors such as age, tumor stage, and histology.
Osteosarcoma
Osteosarcoma develops in new tissue of growing bones, often in the knees, upper legs, and upper arms. It is most common in adolescents and in adults over 65 years of age (Gurney et al., 1999b). Approximately 400 cases are diagnosed every year in the United States in persons younger than age 20, which translates into 5.3 cases per million children under 20 years of age6 (Gurney et al., 1999b).7 In humans, established risk factors for osteosarcoma include occupational or therapeutic exposure to radiation and certain hereditary syndromes (Fuchs and Pritchard, 2002). Studies in animals have shown that exposure to certain chemicals (methylcholanthrene, beryllium oxide, zinc beryllium silicate) and viruses (Rous sarcoma virus, FBJ virus, SV40) can increase the risk of osteosarcoma.
The most common symptom is pain in the affected area. Other symptoms include weight loss, fever, fatigue and anemia. The primary treatment is surgery to remove the affected part of the bone, sometimes requiring amputation of the affected limb. Chemotherapy and surgery are used depending on the stage, location, and size of the cancer. During the period 1985–1994, 63% of children diagnosed with osteosarcoma survived for at least 5 years (Gurney et al., 1999b).
Ependymoma
Malignant ependymomas are a rare type of brain cancer, occurring primarily in children. Incidence is highest in children younger than 3, with a rate of 8.6 per million. Incidence decreases to 1.4 per million in children 5 to 14 years of age8 (Gurney et al., 1999a). An apparent increase from the 1970s to the 1980s in the incidence of brain cancers in children has been attributed by some to improved diagnosis as a result of the increased availability of magnetic resonance imaging (Smith et al., 1998). A small portion of brain cancers in children have been linked to hereditary conditions, but other risk factors are not well established.
Tumors begin in the ependyma, the cells that line the ventricles of the brain, and can occur in either the lower or upper parts of the brain. As a tumor grows, it can obstruct the flow of cerebrospinal fluid through the brain and spinal cord. Ependymomas can metastasize via the cerebrospinal fluid to other areas of the brain and spinal cord. Treatment generally involves surgery to remove as much of the tumor as possible, followed by radiation therapy. Overall, 56% of children with malignant ependymomas survive for at least 5 years; survival rates are higher for older children (Gurney et al., 1999a).
Non-Hodgkin’s Lymphoma
NHL develops within the lymphatic system and includes subtypes of B-cell and T-cell lymphomas.9 Unlike the other three cancers discussed, NHL is more common in the United States, with an estimated 55,000 new cases diagnosed in 2000 (Baris and Zahm, 2000). This translates to an incidence rate for all ages of 19.4 per 100,000. Incidence is low in children and rises steadily with age to 83.4 per 100,000 for adults over 65 years of age10 (Ries et al., 2002). The incidence of NHL has been increasing worldwide, and since the 1970s has increased in the United States at an average annual rate of more than 3% (Ries et al., 2002).
Because immunosuppression is an established risk factor for NHL, a portion of the increase in the incidence of NHL is linked to the spread of HIV/AIDS. However, most of the increase remains unexplained. In addition to primary and acquired immunosuppression, other known or suspected risk factors include genetic factors, infections with certain pathogens (i.e., Epstein-Barr virus, hepatitis C virus, human herpes virus 8, and Helicobacter pylori), some medical treatments, and occupational exposure to pesticides and organic solvents.
The most common and often first symptom of NHL is a painless swelling of the lymph nodes within the neck, underarm, or groin. Other symptoms include, fever, night sweats, fatigue, weight loss, reddened patches on the skin, and itchy skin. The three primary treatments for NHL are radiation, chemotherapy, and immunotherapy, with additional therapies such as bone marrow transplants and peripheral blood stem cell transplantation being explored through clinical trials. The current 5-year survival rate is estimated to be 55% (Ries et al., 2002).
SCIENTIFIC ASSESSMENT
Causality
For its review of the epidemiologic evidence on the association between exposure to polio vaccines containing SV40 and cancer, the committee found studies examining cancer incidence or mortality. Also included in the committee’s review are studies of cancers occurring in children who may have had a prenatal exposure to SV40 through vaccination of their mothers.
In examining the possible association between cancers and exposure to SV40-containing polio vaccines, the committee reviewed several ecologic studies. In an ecologic study, the unit of analysis is a group. Because the data on exposure and disease are available only on a group level, it is difficult to make any causal inferences regarding the association between an exposure and disease at the individual level (Kleinbaum et al., 1982). Thus, although ecologic studies can make only a limited contribution to assessments of causality, only a few other epidemiologic studies of cancer and exposure to the contaminated polio vaccine are available. In contrast to the practice in the committee’s previous reports, the ecologic studies are summarized in the appropriate evidence tables (Tables 1 and 2).
TABLE 1
Evidence Table: Exposure to Polio Vaccine Containing SV40 and Incidence of Cancer.
TABLE 2.
Evidence Table: Exposure to Polio Vaccine Containing SV40 and Cancer Mortality.
The available studies are reviewed in following three categories: cancer incidence, cancer mortality, and cancers following prenatal exposure to SV40-containing vaccine.
Cancer Incidence
Ecologic Studies
United States. Three published studies relied on data from the SEER Program of the National Cancer Institute to examine trends in cancer incidence rates in relation to exposure to SV40 in polio vaccines between 1955 and 1963. The SEER database provides population-based data on cancer incidence and survival assembled from several participating locations in the United States. These locations, which account for about 14% of the U.S. population (NCI, 2002), were chosen to be representative of the U.S. population as a whole. The program began in 1973.
Fisher and colleagues (1999) used SEER data to examine the incidence of cancer at ages 18 to 26 in two U.S. birth cohorts. The cohort born between 1955 and 1959 was considered exposed to SV40. The cohort born between 1963 and 1967 was considered unlikely to have been exposed to SV40 from contaminated polio vaccine. The size of the population at risk was defined as the number of persons ages 20–24 in 1979 (n=2,013,344) and in 1987 (n=1,872,998) for the exposed and unexposed cohorts, respectively.
The incidence of all cancers was 11.0% lower in the exposed cohort than in the unexposed cohort (a decreased risk of 3.7 cases per 100,000 per year) but was 19.6% higher for ependymoma and choroid plexus tumors (an increased risk of 1.6 cases per 10,000,000 per year), 8.4% higher for other brain cancers (a increased risk of 1.7 cases per 1,000,000 per year), 9.76% higher for osteogenic sarcoma (an increased risk of 2.6 cases per 10,000,000 per year), and 178% higher for mesothelioma (based on 6 mesothelioma cases in the exposed cohort and 2 cases in the unexposed cohort; an increased risk of 2.2 cases per 10,000,000 per year). To allow for the effect of other unrelated factors that might contribute to an increase in the background rates of cancer incidence in the unexposed cohort, the authors calculated an adjustment factor by comparing the incidence of all cancers in 1979 for the exposed cohort with the incidence of all cancers in 1987 for the unexposed cohort. They found a 13% difference, which was used to adjust downward the incidence rates for the unexposed cohort.
The authors note that the study is limited by the assumptions regarding exposure and potential biases from diagnostic and coding errors. The committee also noted the lack of formal statistical inference results such as confidence intervals and hypothesis testing, and the imprecise nature of an adjustment that applies a value derived from all cancers to specific cancers.
In a brief report, Strickler and colleagues (1999) used data from SEER and from the Connecticut Cancer Registry to assess trends in the incidence of medulloblastoma in relation to exposure to SV40 through polio vaccine. The SEER data were analyzed using three exposure cohorts: persons born in the period 1956–1962 were defined as exposed to SV40 as infants, those born in 1947–1952 were defined as exposed as children, and those born in 1964–1969 were defined as not exposed. For the exposed cohorts, these data include only cases that occurred at age 9 or older. Compared with the unexposed cohort, the relative risk of medulloblastoma for those exposed as infants was 0.742 (95% CI, 0.55–1.00), and the relative risk for those exposed as children was 0.565 (95% CI, 0.34– 0.94). The paper does not report the incidence rates or the number of cases on which the analysis was based.
The data from the Connecticut Cancer Registry include cases occurring at all ages. Age-specific rates calculated for 5-year periods from 1950–1954 through 1965–1969 showed no trend related to the distribution of the contaminated polio vaccine. For children 0–4 years of age, a non-significant increase occurred after the introduction of the vaccine (1950–1954 compared with 1955– 1959; p=0.16), but rates for 1960–1964 and 1965–1969 (during and after distribution of the vaccine) were similar to those for 1950–1954. The authors noted that since the latency period for cancers in young children is short and the cumulative exposure to SV40 was the greatest at the end of the contamination period, they expected the incidence of medulloblastoma to be the greatest during 1960– 1964, which was contrary to the observed results. Weaknesses in the study include analysis of a single tumor type that may or may not be related to SV40 and an unknown number of cases in the exposed and unexposed cohorts.
In a previous study, Strickler and colleagues (1998) used SEER data to examine trends in ependymoma, osteosarcoma, and mesothelioma in relation to presumed exposure to SV40 in polio vaccines. Age-specific cancer incidence rates for three birth cohorts were compared. As in the previously described study, persons born in 1947 through 1952 were considered exposed to SV40-containing polio vaccine as children, persons born in 1956 through 1962 were considered exposed as infants, and persons born in 1964 through 1969 were considered unexposed. Incidence rates among all three cohorts were based on a total of 200 cases of ependymoma, 522 cases of osteosarcoma, and 71 cases of mesothelioma.
Using a Poisson regression model and controlling for age, no significant elevation in cancer incidence or cancer risk was found for either of the exposed cohorts when compared with the unexposed cohort. The risk in the cohort exposed as infants relative to that in the unexposed cohort was 1.06 (95% CI, 0.69– 1.63); the risk for those exposed as children compared with the unexposed was 0.98 (95% CI, 0.57–1.69). For all brain cancers, the relative risk was 0.90 (95% CI, 0.82–0.99) for the cohort exposed as infants and 0.82 (95% CI, 0.73–0.92) for the cohort exposed as children. Because SEER began in 1973, data are not available to evaluate the incidence of ependymoma in the exposed cohorts at ages less than 5 years, when incidence is highest. Data for 1950–1969 from the Connecticut Tumor Registry showed 22 ependymomas in children under age 5. The authors noted that incidence was higher for the period spanning 1950–1954, before IPV was in use, than for the period spanning 1960–1964.
For osteosarcoma, the SEER data cover teenagers and young adults, age groups that are at increased risk for this cancer. Compared with the unexposed cohort, the relative risk for osteosarcoma for the cohort exposed to SV40 as infants was 0.87 (95% CI, 0.71–1.06) and was 0.85 (95% CI, 0.59–1.22) for the cohort exposed as children. The relative risk for mesothelioma was 3.00 (95% CI, 0.67–13.11) for those exposed as infants and 2.45 (95% CI, 0.50–12.03) for those exposed as children. The authors note that the cohorts studied have not yet reached ages at which the incidence of mesothelioma is highest.
Overall, the committee found the studies by Strickler and colleagues (1999, 1998) to be more rigorous methodologically than the study by Fisher and colleagues (1999). However in addition to the weaknesses noted above, all three studies have limitations. First, the SEER data are subject to misclassification bias because of changes over time in coding practices and in diagnostic criteria. Second, it is not precisely known how many people in any of these cohorts received polio vaccine that contained SV40. Third, the SEER data would be biased to the null if it covers states that did not receive the contaminated vaccine. These weaknesses limit the contribution that the studies make to the committee’s assessment of causality.
Sweden. A presentation by Olin and Giesecke (1998) was reported in the proceedings of a 1997 NIH conference on SV40 (Brown and Lewis, 1998). The authors examined differences in the incidence of brain cancers, ependymoma, osteosarcoma, and pleural mesothelioma in Sweden among cohorts exposed and unexposed to polio vaccine containing SV40. Polio vaccine believed to have contained SV40 was administered in 1957 to children born between 1946 and 1953. Vaccination records showed that 70.4% of the children born between 1946 and 1949 and 59% of the children born between 1950 and 1953 received the vaccine. Vaccine used since 1958 was reportedly free of SV40. Data on cancer incidence from 1960 through 1993 were obtained from the Swedish National Cancer Registry.
For each cancer considered, the authors reported age-adjusted incidence rates for men and women for 5-year periods from 1960 through 1990 (e.g., 1960–1964, 1965–1969, etc.). They also calculated relative risks (exposed versus unexposed) for the incidence of each of the cancers for several 5-year age groups (i.e., 10–14 through 40–44). The incidence data for the exposed group were based on the 3 consecutive calendar years with the highest proportion of exposed persons in a given age group. For the 35–39 age group, for example, the data for the exposed population come from 1985–1987. The data for the unexposed 35- to 39-year-olds are from 1980 and 1993, the closest years in which none of the exposed population was in the 35–39 age group. The authors conclude that the incidence of these cancers was similar in the exposed and unexposed populations.
This report makes a weak contribution to the committee’s assessment of causality. Although relative risks for the exposed versus the unexposed cohorts were reported, no confidence intervals were provided. In addition, the relative risks were not consistent across age groups and no statistical tests for trends were performed. Misclassification bias is possible because vaccine-based exposure to SV40 remains uncertain.
Germany. Geissler (1990) reports data from the National Cancer Registry in the German Democratic Republic on the frequency of tumors in two cohorts after a 22-year follow-up. An exposed cohort was defined as individuals born in 1959–1961 (n=885,783), of whom more than 86% received Sabin-Chumakov oral polio vaccine presumed to have contained SV40. An unexposed cohort was defined as individuals born in 1962–1964 (n=891,321) who received polio vaccine free of SV40. The exposed group had fewer astrocytomas, ependymomas, meningiomas, neurinomas, retinoblastomas, other brain tumors, hemoblastoses, sarcomas, other malignancies, and preneoplasias than the unexposed group, and more gliomas and glioblastomas, medulloblastomas, oligodendrogiomas, plexuspapillomas, spongiblastomas, melanomas, and Wilms tumors. Overall, the incidence of tumors was 28.7 per 10,000 in the exposed group and 30.1 per 10,000 in the unexposed group.
With only the frequencies of tumors reported for each cohort, the rarity of some of the tumors, and no relative estimates or statistical analysis, it is difficult to evaluate the magnitude of the differences between the two cohorts and any relationship between cancer incidence and exposure to polio vaccine containing SV40. The study’s contribution to causality is further weakened by the evidence that exposure to contaminated OPV, in contrast to IPV exposure, does not produce an antibody response to SV40 (as reviewed in Shah and Nathanson, 1976).
Controlled Observational Studies
The committee also reviewed two early case-control studies that examined the oncogenic potential of the polio vaccine. These studies have critical limitations, however. They do not meet current epidemiologic standards for analysis since they do not provide risk estimates or confidence intervals to estimate the strength of the association between polio vaccination and cancer. They also lack specificity in terms of the health outcomes used to define the cases. They examine malignancies in general or types of malignancies that are not currently linked to SV40 (e.g., leukemia). Finally, these study reports do not discuss the likelihood that the study subjects received polio vaccine contaminated with SV40. Overall, these studies contribute little to understanding causality but are summarized here because they are the only controlled observational studies examining the association between exposure to the polio vaccine and cancer.
Australia. Innis (1968) compared the immunization histories of 816 pairs of children with and without cancer who were admitted to hospitals in Sydney and Brisbane, Australia, between January 1958 and May 1967. Controls were matched according to sex, hospital, and age. Controls in Sydney were selected from a pool of cases admitted before and immediately after children suffering from leukemia, cancer, or ulcerative colitis. In Brisbane, controls were chosen by random selection of charts until an appropriate age and sex match were found. A child’s immunization history was determined from the immunization status recorded in the medical record by a medical attendant at the time of admission. Immunizations considered in the study included those for polio, diphtheria, pertussis, tetanus, smallpox, BCG, typhoid, and cholera. Among the children under 1 year of age (n=110 pairs), 29 cases and 28 controls had been exposed to polio vaccine. Among children over 1 year of age (n=706 pairs), 618 cases and 569 controls had received polio vaccine. This difference was significant (chi-square=12.182; p<0.0005). The immunization status of cases and controls was comparable for other vaccines.
Assessment of the potential contribution of SV40 to the incidence of cancer is limited by a lack of information on the history of SV40 contamination in polio vaccine used in Australia and on the timing of polio vaccination for cases and controls.
United Kingdom. In a research letter, Stewart and Hewitt (1965) reported on exposure to polio vaccine among children with cancer and their paired controls. Comparisons were made for 999 cases of leukemia in children up to 9 years of age at death and their matched controls and for 1,108 children with other malignant disease and their matched controls. Exposure to polio vaccine was reported in 270 cases of leukemia and in 259 controls. For other malignant disease, 259 cases and 265 controls were exposed to polio vaccine. Information was also reported on exposure to other vaccines, specifically, diphtheria, pertussis, smallpox, tetanus, BCG, yellow fever, and typhoid.
Since only the number of cases is reported, it is difficult to assess the significance of these differences and the strength of any association between exposure to polio vaccine and cancer. The likelihood of exposure to SV40 in polio vaccine is not discussed.
Cancer Mortality
Ecologic Studies
United States-Brain Cancer. Strickler and colleagues (1998) compared mortality from brain cancer among children under age 5 in three birth cohorts. Children born in 1947 through 1952 did not receive polio vaccine until after age 5 and so were not considered exposed to SV40 for purposes of this analysis. Children born in 1956 through 1962 were considered exposed to SV40 as infants, and children born in 1964 through 1969 generally received polio vaccine as infants but were considered unexposed to SV40. Mortality data were obtained from the National Center for Health Statistics (NCHS).
Brain cancer mortality showed no association with exposure to polio vaccine containing SV40. The mortality rate in the cohort that did not receive polio vaccine by age 5 was 2.04 per 100,000 person-years, compared with 1.27 per 100,000 person-years in the SV40-exposed cohort and 1.04 per 100,000 person-years in the cohort that received SV40-free polio vaccine. The rates were reported to be significantly different from each other, based on a total of 4,643 deaths and 333,163,427 person-years of observation.
United States-All Cancers and Leukemia. Fraumeni and colleagues (1963) examined cancer mortality rates from 1950 through 1959 to assess the effect of exposure to SV40 containing polio vaccines. Use of polio vaccine began in 1955 and was initially targeted to children in first and second grades (approximately 6 to 8 years old). Additional groups were vaccinated as the supply of vaccine increased. By 1957, an estimated 60 million people under 50 years of age (47%) in the civilian, noninstitutionalized population had received at least one dose of vaccine. This included 45 million persons under 20 years of age (72% of that age group). Mortality data for ages 0 to 24 years for leukemia, all cancers except leukemia, and selected sites (brain, kidney, and connective tissue) were obtained from published vital statistics.
From 1955 to 1959, leukemia mortality increased from 3.5 to 3.8 per 100,000 in children 5 to 9 years of age and from 2.2 to 2.5 per 100,000 in children 10 to 14 years of age (for each age group, an increase of 3 deaths per 1,000,000). For all other cancers and for brain, kidney, and connective tissue cancers, there were only minor fluctuations in mortality rates. The authors noted that the study would be unable to detect an effect on the incidence of cancers with a latency period of more than 4 years.
Fraumeni and colleagues (1963) also compared incident cancer rates in children who received the polio vaccine at 6–8 years of age in May-June 1955. Cancer mortality data for 1950 through 1959 for this cohort of children were obtained from the National Vital Statistics Division, and mortality rates were calculated using detailed population estimates. Analysis of stored samples of the vaccine administered to these children showed variations in the level of SV40 contamination. Estimates of per capita doses of SV40 were used to define three groups of states according to the level of contamination in the vaccine administered; states were classified as high-level SV40, low-level SV40, and no SV40.
In general, the mortality rates peaked in 1951–1952 and then decreased through 1959, when the study ended. Mortality rates were higher both before and after vaccination began in states that had contaminated vaccine than in states with SV40-free vaccine. The authors noted that the difference might have been due to geographic variations in matters such as cancer reporting and racial/ethnic make-up of the population. Rates of decline were similar for the SV40 states and the non-SV40 states after 1955.
The lack of formal statistical inference results of differences and trends and of information on variances limits the contribution of this study to an assessment of causality.
Uncontrolled Observational Studies
Follow-up study. Between 1960 and 1962, polio vaccine inadvertently containing SV40 was administered within 3 days of birth to 1,077 infants at the Cleveland Metropolitan General Hospital in a study to assess the feasibility of immunization in the presence of maternal antibodies (Fraumeni et al., 1970). According to medical records, 925 children received monovalent OPV, some of which contained high titers of SV40, and 152 children received IPV, which had lower concentrations of SV40. Later in infancy, children received booster doses of attenuated or inactivated polio vaccine, or both, which presumably contained SV40. Reports of follow-up studies when the children were 8 years old (Fraumeni et al., 1970), 17–19 years old (Mortimer et al., 1981), and recently at 41–42 years of age (Carroll-Pankhurst et al., 2001) have also been published.
No cancer deaths were identified in the first two follow-up studies, compared with one expected death on the basis of age-specific rates for the United States (Mortimer et al., 1981). In the most recent study (Carroll-Pankhurst et al., 2001), deaths in the study population were ascertained through the National Death Index. A total of 44 deaths were identified, including 4 cancer deaths (two each for leukemia and testicular cancer). Expected numbers of deaths were estimated from NCHS rates for Cuyahoga County, Ohio, the location of the original immunization study. The relative risk for observed versus expected cases was 36.98 (95% CI, 4.47–133.50) for testicular cancer, 4.2 (95% CI, 0.1–15.73) for leukemia, and 1.26 (95% CI, 0.34–3.23) for all cancers. The authors observed that testicular cancer has not previously been linked to SV40 and that the excess risk found in this study may well be due to chance. There were no deaths from brain tumors, osteosarcoma, or mesothelioma, the cancers linked to SV40 exposure in other studies. Overall, the study is limited by the small number of subjects and possible bias from deaths missed and or not identified by the National Death Index. In addition, the duration of follow-up may still be insufficient to see any effect on mesothelioma.
Prenatal Exposure to Polio Vaccine Containing SV40
Controlled Observational Studies
Collaborative Study on Cerebral Palsy, Mental Retardation and other Neurological and Sensory Disorders of Infancy and Childhood. Heinonen and colleagues (1973) compared cancer incidence rates in children born to mothers who, between 1959 and 1965, received the killed polio vaccine (n= 18,342), the live attenuated oral vaccine (n=3,056), or no polio vaccine (n= 32,555). The study population was drawn from pregnant mothers participating in the Collaborative Study on Cerebral Palsy, Mental Retardation and other Neurological and Sensory Disorders of Infancy and Childhood. The mothers’ immunization histories (killed polio vaccine, attenuated live polio vaccine, and influenza vaccine) were determined through periodic interviews during pregnancy and from review of hospital records. Information on the incidence of malignancies was available for children up to one year of age. Mortality and autopsy information were available for children up to 4 years old.
At the time the data were analyzed, malignancies had been detected in 24 children. The malignancies included neurologic tumors (n=8), leukemia (n= 8), renal tumors (n=6), granulose cell tumors in an ovotestis (n=1), and hepatoblastoma (n=1). Malignancies in nine of these children were diagnosed at autopsy, and four occurred in premature infants who died within 24 hours of birth. Three of these malignancies (2 adrenal neuroblastoma, 1 nephroblastoma) were diagnosed using microscopic methods.
Of the 24 children with malignancies, 14 were born to mothers who received the killed polio vaccine during pregnancy (7.6 per 10,000), and the rest were born to mothers who were not vaccinated (3.1 per 10,000). The difference between the two rates is statistically significant (p<0.05). For neurologic tumors, the rate of 3.8 per 10,000 in the exposed group was significantly higher (p <0.01) than the rate of 0.3 per 10,000 in the unexposed group. The differences in rates for leukemia and for other malignancies were not statistically significant. No malignancies occurred among the children of mothers who received the oral polio vaccine.
The limitations in the study, such as the small number of cancer cases, and the weaknesses, such as the potential for misclassification bias related to exposure and to the identification of some tumors at autopsy that might never have progressed to overt disease, limit the study’s contribution to the assessment of causality.
Rosa and colleagues (1988) reported on additional analyses from this study. Stored serum samples from the mothers of the 24 children with malignancies and from matched controls were tested for SV40 antibodies. Controls were matched for institution, maternal age, race, and last menstrual period. Two serum samples, taken at entry into the study and at delivery, were available for each woman. The serum from the mothers of the eight infants with neurologic tumors was negative for antibodies to SV40. Two mothers of infants with leukemia tested positive for SV40 antibodies, but only one of them showed sero-conversion during pregnancy. The authors also report that 3 of 36 controls had antibodies to SV40 in both serum samples. They suggest that the association between neurologic tumors and administration of IPV reported by Heinonen and colleagues (1973) was not related to SV40.
Connecticut Tumor Registry. In a study by Farwell and colleagues (1979), records of the Connecticut Tumor Registry were reviewed to identify children who were born between 1956 and 1962 and who had developed neoplasms of the central nervous system (CNS) by age 19. From a total of 120 children, 40 cases were randomly selected. The cases included gliomas (astrocytoma, spongioblastoma, and glioblastoma multiforme) and medulloblastomas. Each case was matched to two controls on the basis of sex, birth date, and birth place. Questionnaires were sent to the obstetricians who delivered the children for information on the mothers’ history of polio vaccination during pregnancy.
The authors reported that among 52 cases of CNS tumors, 19 (37%) had a history of maternal polio immunization and presumed exposure to SV40. In comparison, 8 of 38 controls (21%) had been exposed. This difference was not significant (p=0.15). However, the committee notes that the basis for a report of 52 cases is unclear, since the study is described as starting with 40 cases (and 80 controls).
Of the 23 children with gliomas, 8 were exposed to polio vaccine presumed to contain SV40. Although no data for the controls were reported, the authors consider the results suggestive of an association with exposure to SV40. Of the 15 medulloblastoma cases, 10 were exposed to SV40. Compared to the controls (again, no data reported), this rate of exposure is significantly higher (p<0.01).
Causality Argument
All of the studies that the committee reviewed concerning cancer incidence or cancer mortality and exposure to polio vaccine containing SV40 have substantial limitations. Many of these studies were ecologic in design. In previous reports, the committee included only controlled observational studies in tables summarizing the evidence. Ecologic studies were excluded based on their inherent limitations in assessing causality. In general, it is difficult to make causal inferences from ecologic studies, mainly because: 1) exposure and outcome data are based on measurements averaged over a population, 2) exposure information is based on proxy measures (e.g., SV40 exposure based on birth cohort), and 3) data to control for confounding are unavailable (Morgenstern, 1998). For this report, however, because of the few controlled observational studies available and the public health importance of the issue at hand, the committee has included all of the studies it reviewed in the relevant evidence tables (Tables 1, 2, and 3).
TABLE 3
Evidence Table: Prenatal Exposure to Polio Vaccine Containing SV40 and Incidence of Cancer.
Most of the epidemiologic studies on polio vaccine containing SV40 and cancer are subject to misclassification bias because they rely on year of birth to designate exposure status. Even though polio vaccine known to contain SV40 was in use from 1955 to 1963, it is difficult to accurately determine which individuals received the vaccine without individual vaccination records. The studies may also be subject to misclassification bias because of lack of detailed and specific information about the presence of SV40 in individual vaccine doses. Information reported by Fraumeni and colleagues (1963) suggests that at least some vaccine used in 1955 was not contaminated. Further, samples of polio vaccine produced during the remainder of the period of likely contamination (1955 to 1961) were not available for testing, leaving little means to identify which vaccine lots were contaminated and who was exposed to SV40 contaminated polio vaccine. In addition, the assumption that persons who received polio vaccine after 1963 were unexposed to SV40 may not be accurate if sources of exposure other than contaminated IPV exist.
The studies were also limited by the rarity of the tumors thought to be associated with exposure to SV40. The effect estimates calculated from a small number of tumors are more sensitive to distortion from confounders, bias, and chance. The cohort exposed to contaminated vaccine has not yet reached the age when the cancers of interest are of high incidence and the associations in particular cannot be ruled out by the evidence to date. Studies of cancer mortality are also subject to confounding due to improvements over time in the effectiveness of treatments, which may produce a decline in mortality rates that is unrelated to the incidence of the cancer.
Even if the associations suggested by some studies in this body of weak epidemiologic evidence are true, the absolute risks for additional cancer cases or deaths are small and cannot necessarily be attributed solely to exposure to SV40-contaminated polio vaccine. For example, the 178% increase in the incidence of mesothelioma reported by Fisher and colleagues (1999) implies an increase of 2.2 cases of mesothelioma per 10,000,000 population per year. The increased mortality risk from leukemia in the report by Fraumeni and colleagues (1963) was 3 deaths per 1,000,000 population.
Based on these limitations, the committee concludes that the evidence is inadequate to accept or reject a causal relationship between SV40-containing polio vaccines and cancer.
Biological Mechanisms
Although biological data do not provide an independent basis for evaluating causality, they can help validate epidemiologically based conclusions for or against causal associations. Such data can also guide further investigation when epidemiologic evidence is inconclusive.
The committee’s task was to consider the evidence regarding the potential for SV40-contamination of polio vaccines in the late 1950s and early 1960s to cause cancer. Concern has focused on the four cancers discussed above: mesothelioma, ependymoma, osteosarcoma, and NHL. The committee reviewed the evidence on biological mechanisms related to this hypothesis through three key questions:
- 1.
-
Is SV40 a transforming virus?
- 2.
-
Can SV40 cause cancer in humans under conditions of natural exposure?
- 3.
-
Is contamination of the polio vaccine with SV40 responsible for SV40 infection in humans?
Given that the epidemiologic evidence regarding a causal relationship was inconclusive, the committee reviewed the biological evidence with an eye towards additional research that might be needed to better understand the putative role that exposure to SV40 from polio vaccines might have in cancer.
A wealth of literature exists on topics such as the presence of SV40 in tumors and the effects of the virus or its gene products (particularly the large tumor antigen, Tag) in cell cultures. Several large scientific conferences have also been held to review progress in understanding the role of SV40 in human cancers. Because the Immunization Safety Review Committee was not charged with resolving the full range of uncertainties about the biology of SV40 and the role of this virus in human cancers, the review that follows provides only highlights of the key arguments on these issues. More detailed discussion is available in several excellent and comprehensive reviews (Brown and Lewis, 1998; Butel and Lednicky, 1999; Carbone et al., 1997b; Klein et al., 2002; Strickler, 2001b).
Is SV40 a Transforming Virus?
Evidence suggesting that SV40 can produce oncogenic transformation of cells comes from four sources: rodents, nonhuman primates, cell culture studies, and humans.
Rodents. The earliest studies of SV40 were conducted with rodents and showed that administration of SV40 to neonatal and weanling hamsters caused cancers. A seminal study (Eddy et al., 1961) demonstrated that injection of extracts of rhesus monkey kidney-cell cultures into newborn hamsters was followed by the occurrence of neoplasms in approximately 70% of the animals. The presence of SV40 in these extracts was confirmed in a subsequent study (Eddy et al., 1962). Histologic examination of the neoplasms determined that they were undifferentiated sarcomas in the subcutaneous tissue, kidneys, and lungs. Infrequent subcutaneous lobules resembled fibrosarcomas. The tumors were noted to be different from those caused by the SE polyoma virus, a mouse virus that also induces tumors in newborn hamsters.
Other studies showed that intracerebral inoculation of neonatal hamsters resulted in ependymomas (Kirschstein and Gerber, 1962) and that intravenous injections of weanling hamsters resulted in the formation of several types of tumors, including lymphomas and sarcomas (Diamandopoulos, 1973). More recent experiments show the development of mesothelioma in hamsters subsequent to SV40 injection (Cicala et al., 1993). Despite the limitations in their applicability to humans, these animal systems are notable in the concordance of three types of tumors with ones that have been associated with the presence of SV40 DNA sequences or Tag—ependymoma, sarcomas (osteogenic), and non-Hodgkin’s lymphoma. Transgenic mice expressing SV40 gene also develop transformed cells and cancers in the cells in which the transgene is expressed (Butel and Lednicky, 1999; Wang and Garcea, 1998).
Nonhuman primates. As noted, macaques that were immunocompromised as a result of SIV-infection have developed central demyelinating disease (Holmberg et al., 1977; Horvath et al., 1992), suggesting CNS migration of SV40. At least one SIV-immunocompromised macaque developed an astrocytoma that was positive for SV40 DNA (Hurley et al., 1997).
Cell-culture studies. Shortly after the identification of SV40, it was established that the virus can transform cultured human cells (Koprowski et al., 1962). It now appears that the transforming properties of SV40 are due, primarily if not solely, to the effects of Tag on key proteins involved in controlling cell growth (Butel and Lednicky, 1999; Kim et al., 1998; Rundell et al., 1998). In particular, Tag inactivates the tumor-suppressor proteins p53 and Rb. These gene products normally suppress tumor growth by preventing inappropriate cell cycling and by promoting the death of cells with genetic damage. By inactivating these proteins, SV40 Tag promotes both transformation and immortalization of cells. There are an abundance of data from cell culture systems demonstrating effects of SV40 or Tag on many steps related to cell transformation. In addition, evidence from cell cultures of human mesothelial cells suggests that SV40 might preferentially infect these cells without lysis (Bocchetta et al., 2000). This could explain effects of SV40 leading to tumors in some tissues and not others.
Humans. Cells transformed by SV40 have been shown to grow in humans and become tumors (Jensen et al., 1964). In this study, persons terminally ill with cancer received implants of either homologous or autologous tissue via subcutaneous injection. When cells transformed by SV40 were implanted, nodules of undifferentiated tumor cells developed. Injection of uninfected cells or of cells infected with SV40 but not yet transformed rarely resulted in the formation of nodules. These nodules regressed 10 to 40 days after implantation, which appeared to reflect immune-mediated rejection of these allogeneic or syngeneic SV40-transformed tumors. This study provides evidence from contrived clinical conditions that cells transformed by SV40 in vitro can develop into undifferentiated tumors in a human host. It also indicates that such tumors are normally eliminated by the host immune response, which occurred even in this group of individuals whose immune systems were likely to have been compromised. However, it is possible that individuals with more profoundly compromised immunity might not have been able to eliminate the tumors.
The committee concludes that the biological evidence is strong that SV40 is a transforming virus.
Can SV40 Cause Cancer in Humans under Conditions of Natural Exposure?
Although there is a theoretical basis for mechanisms by which SV40 could cause cancer in humans and mechanistic evidence is accumulating to support the theory, it is not clear which specific mechanisms might be operable. The principal lines of evidence for the operation of specific mechanisms are that SV40 acts in ways consistent with tumorigenesis and that DNA sequences consistent with SV40 have been detected in several types of human tumors.
Evidence that SV40 could be tumorigenic comes from in vitro studies and studies in animals. These studies, some of which were reviewed above, point to the critical role of SV40 Tag, which is found in the nuclei of transformed cells. As noted, substantial evidence suggests that Tag binds and inactivates the products of tumor-suppressor genes, especially the p53 and Rb proteins. The inactivation of these proteins allows for unregulated cell division (Butel and Lednicky, 1999; Klein et al., 2002). Because continuous expression of Tag is required to maintain a transformed cell line in hamsters (Carbone et al., 1997b), the failure to detect SV40 Tag in some human tumors of interest has been cited as a counter argument to a tumorigenic role for SV40 (Strickler, 2001b). However, studies with transgenic mice and with human cells in culture appear to suggest that under certain circumstances, SV40-induced cell transformations can persist with little or no expression of Tag (Carbone et al., 1997b), presumably reflecting the development of additional transforming mutations or genetic instability as a result of earlier abolition of cell cycle checkpoint fidelity by Tag.
Data on the association between SV40 and human tumors are inconsistent. A growing body of clinical studies reports the detection of SV40 DNA in several types of tumors. The most notable and well-studied of these is mesothelioma (Carbone et al., 1999, 2002). In addition, SV40 DNA has been detected in bone cancers (Carbone et al., 1996), ependymomas (Bergsagel et al., 1992; Lednicky et al., 1995), and in non-Hodgkin’s lymphoma (Shivapurkar et al., 2002; Vilchez et al., 2002). Other studies, however, report an inconsistency or absence of SV40 in mesotheliomas, osteosarcomas, and brain tumors (Engels et al., 2002; Heinsohn et al., 2000; Strickler, 2001a; Strickler et al., 1996). Shown in Table 4 are the reports from some studies in which mesotheliomas, the tumor in which SV40 has been most strongly implicated, were evaluated for the presence of SV40 by a variety of techniques, most commonly by PCR. Due to the potential for PCR to detect sequences from other polyoma viruses found in humans (BK and JC), in most studies the investigators used one or more tests to enhance the specificity for SV40, the most stringent of which is sequencing of the PCR products. Most studies report the detection of SV40 sequences in some of the mesotheliomas, but few also examined normal tissues in parallel. In some cases, the presence or absence of SV40 in tumors was attributed to geographic differences in exposure to SV40 (e.g., in Finland and Turkey, SV40 containing polio vaccines were never used) or to the multifactorial nature of some cases (DeRienzo et al., 2002; Hirvonen et al., 1999; Reuther et al., 2001). The association of Epstein-Barr virus infection with Burkitt’s lymphoma in Africa but not in European countries provides a precedent for geographic variability in outcomes. When SV40 viral fragments or Tag is detected in a tumor sample, it is often present in only a fraction of the cells. Although EBV is reported in only a portion of tumor cells with which it is associated (Grinstein et al., 2002), HPV is found in all cells of the cervical tumors.
Table 4.
SV40 Sequences in Human Mesothelioma.
The conflicting results in the detection of SV40 have also led to questions about technical aspects of the detection of the virus. It is unclear whether positive findings are the result of overly sensitive but nonspecific tests that are detecting other viruses (i.e., BK or JC) or SV40 from laboratory contamination, or whether negative findings arise from a lack of sensitivity in the detection methods used. Two multicenter studies have attempted to resolve some of the uncertainty regarding the detection of SV40 in human mesothelioma samples.
The first of these studies (Testa et al., 1998) was conducted with the goals of determining the presence or absence of SV40 in mesothelioma samples and investigating whether a correlation exists between SV40 and asbestos exposure.11 Participating in the study were four independent laboratories, including one that works with the BK and JC viruses, but not with SV40. Twelve frozen mesothelioma samples, along with positive and negative controls, were analyzed for the presence of SV40 DNA with two different PCR primer sets. Positive results were confirmed by Southern blot hybridization and by DNA sequencing of the PCR products. For 9 of the 12 samples, the results from each of the four laboratories were positive for the presence of SV40 DNA. But the laboratories’ results for the other three samples were inconsistent. The positive and negative controls gave consistently appropriate results. Further study with immunohistochemical techniques in one of the laboratories found that 10 of the 12 samples, including the 9 that were consistently positive for SV40 DNA, contained cells that were positive for SV40 Tag. The authors could not account for the inconsistencies in the detection of SV40 DNA in three of the samples, but they suggested that those samples might have had a smaller proportion of cells with SV40, compared with the other samples.
The formal goals of the second multicenter study were to compare methods for the detection of SV40 DNA, to examine the ability of these methods to detect SV40 in human mesothelioma, and to understand possible reasons for the conflicting findings in past investigations (Strickler, 2001 a). Each of the nine participating laboratories received masked, paired replicate DNA samples from 25 fresh-frozen mesothelioma specimens and one DNA sample from each of 25 normal lungs. Masked positive and negative controls were also included, but contamination of the initial set of negative control samples by the central processing laboratory led to a number of false-positives. Although the problem of falsely positive negative controls was largely, but not completely, eliminated by the generation of a second set of control samples, this raises concerns in the minds of some regarding study reliability. SV40 was detected in both paired samples from one of 25 mesothelioma specimens in one of the laboratories and from a different one of the 25 mesotheliomas in another laboratory. The other participating laboratories did not detect SV40 in either of these two samples, and either did not detect SV40 DNA in any sample or did so on only one of the two replicates of one of the other 25 mesothelioma samples. Because these collaborative efforts failed to resolve why some laboratories detect SV40 and others do not, the presence, specificity and strength of the association between SV40 and certain types of human tumors remain uncertain.
The detection of SV40 in tumors does not, by itself, demonstrate a causal relationship. SV40 could be a passenger virus, infecting the cells but causing no pathology. Findings from studies examining SV40 in mesothelioma (Table 4) demonstrated a great deal of variability, which preclude the ability at present to draw firm conclusions regarding the frequency with which SV40 can be detected in specific neoplasms and/or normal tissues in humans. Some studies have detected SV40 in a variety of normal tissue, blood and cells shed into the urine from healthy subjects (Li et al., 2002; Martini et al., 1996; Woloschak et al., 1995). Its detection in multiple types of tumors indicates a lack of specificity for a single type of cancer, and also leads to doubts about a causal link (Strickler, 2001b). Although not unheard of, infection of such a broad range of tissues is unusual. In healthy rhesus monkeys, SV40’s natural host, the virus infects primarily kidney cells. HPV, a related papovavirus, has been linked only with a single type of tumor—cervical cancer. However, some viruses are associated with more than one type of tumor. Epstein-Barr virus, for example, is associated with Burkitt’s lymphoma and nasopharyngeal cancer. Additionally, because one of the receptors for a SV40 gene product is a widely distributed MHC protein, effects on multiple tissue types might be expected.
The committee concludes that the biological evidence is moderate that SV40 exposure could lead to cancer in humans under natural conditions.
Is Contamination of the Polio Vaccine with SV40 Responsible for SV40 Infection in Humans?
Although it is incontrovertible that some polio vaccine was contaminated with SV40, the nature and extent of human exposure to SV40 through this or other sources is less clear. In the United States, potentially contaminated IPV was administered between 1955 and 1963. As noted, tests of samples of the vaccine that had been used in 1955 showed that the levels of contamination varied and that some vaccine was not contaminated (Fraumeni et al., 1963). Samples of vaccine used between 1956 and 1963 were not tested, and no documentation is available to identify specific lots or vials of IPV that were contaminated or the amount of live SV40 that was present in any contaminated vaccine. One estimate, based on the tests of the 1955 vaccine, suggests that 10 to 30 million people of the 98 million who were vaccinated with IPV before 1963 could have been exposed to live SV40 (CDC, 2001; Shah and Nathanson, 1976).
Serologic tests have shown the presence of antibodies to SV40 in children likely to have received contaminated IPV (Gerber, 1967; Shah et al., 1972). While these results confirm exposure to SV40, they do not distinguish between exposure to live and killed SV40. Because the process for inactivation of the live polio virus could be expected to have also killed some of the SV40, some vaccinees are likely to have been exposed to a mixture of the live and killed virus and others only to killed SV40. The presence of antibody might also indicate protective immunity against SV40-induced oncogenesis. Thus, exposure to IPV between 1955 and 1963 cannot be equated with exposure to, or infection with, SV40 and it is unknown which and how many individuals became infected with SV40 through the receipt of contaminated IPV.
OPV used for clinical trials (1958–1959) in the United States prior to its licensure in 1960 was also contaminated with SV40. The level of contamination was high, but exposure was more limited, with approximately 10,000 people thought to have received vaccine from contaminated lots. Studies showed, however, that the recipients of contaminated OPV produced no antibody response to SV40 (reviewed in Shah and Nathanson, 1976), indicating less likelihood of infection through oral exposure. This suggests that IPV but not OPV resulted in the infection of humans with SV40. Nonetheless, concerns about the validity, and in particular the specificity for SV40, of the serological testing creates some uncertainty about this conclusion. Though the suggestion has been made (Kops, 2000) that some OPV administered after 1963 might also have contained SV40, there is not evidence to support this. In three studies that sought to detect SV40 in OPV, SV40 was not found (Rizzo et al., 1999; Sangar et al., 1999; Sierra-Honigmann and Krause, 2000).
Additional uncertainty about the possible contribution of vaccine-based SV40 exposure to SV40 infection and carcinogenesis has arisen because of the age at which vaccinees were exposed. Carcinogenicity in rodents is essentially limited to animals exposed as neonates or weanlings—when they are immunologically immature (Diamondopoulos, 1973; Eddy et al., 1961, 1962; Kirchstein and Gerber, 1962). If this model holds in humans, then school-aged children, adolescents and adults who received contaminated IPV would likely have been at less risk for any carcinogenic effects than those who received the vaccine at younger ages, and in particular, those who might have been exposed in the first half of gestation through infancy, ages at which immunologic competence approximates that of rodent neonates and weanlings respectively (Lewis and Wilson, 2000; Siegrist, 2001). Because the incidence of ependymomas is highest in children under age 5 years and osteosarcoma is most common in adolescents, contemporary evidence of SV40 in such tumors does not provide a direct link to exposure to contaminated IPV between 1955 and 1963. But with the long latency period for mesothelioma and the risk for non-Hodgkin’s lymphoma being greatest in older individuals, contaminated IPV remains a possible source of SV40 exposure in this population.
Polio vaccine from 1955 was recently discovered and analyzed. It was found to contain a strain of SV40 with only one 72-bp enhancer in the regulatory region (Rizzo et al., 1999). This differs from the SV40 originally identified from polio vaccine, which had 2 72-bp enhancers, and is similar to that found in some of the human tumors reported associated with SV40 (Vilchez et al., 2002).
Human exposure to SV40 occurred through contaminated IPV and OPV, but other sources of exposure to SV40 may also exist. A limited number of people are known to have been exposed to SV40 through other vaccines. These include an experimental live-virus vaccine against respiratory syncytial virus, used in 1956, and a licensed inactivated adenovirus vaccine that was administered to military recruits between 1955–1961 (Shah and Nathanson, 1976). Evidence of SV40 exposure has also been detected in serologic surveys done before 1955. In a study of sera collected prior to polio vaccination from medical students in Wisconsin in 1952, IgG against the capsid antigen of SV40 was detected by ELISA in 6 of 51 samples tested (Geissler et al., 1985). Other studies of people not exposed to the contaminated polio vaccine also showed evidence of antibodies to SV40 that were not obviously due to cross-reactivity to BK or JC viruses (Shah et al., 1972). However, as has been discussed, serologic detection of SV40 infection is problematic and it is hard to determine whether these early samples, particularly those from before 1955, do or do not support infection with SV40.
Detection of SV40 in persons too young to have received contaminated polio vaccine suggests the possibility of continuing transmission of SV40 through means other than the polio vaccine. Possible sources of exposure to SV40 are person-to-person transmission, animal-to-person transmission, and laboratory exposure to SV40. Transmission from animals (macaques) to persons is well documented (Shah, 1972), as is exposure in laboratory workers. SV40 is a common research tool in many laboratories, providing a route of exposure for some people too young to have received the contaminated polio vaccine. Evidence of person-to-person transmission is less clear. Among monkeys, SV40 shed in the urine of infected animals is thought to be a primary means of viral transmission (Butel and Lednicky, 1999). Similarly, the BK and JC viruses are probably transmitted by viral shedding in the urine of infected persons. But SV40 is rarely detected in human urine, even in immunocompromised persons (Bofill-Mas et al., 2000; Shah et al., 1997). However, in one recent study, cells harboring infectious SV40 were detected in the urine in a substantial fraction (>10%) of persons (Li et al., 2002). While these results suggest exposure to SV40, there is some uncertainty regarding the specificity of the serologic testing. Furthermore, the serologic tests do not clearly distinguish between exposure to live or killed SV40.
Finally and perhaps most importantly, measures of exposure remain problematic. The serology data are unclear, in part because of concerns about cross-reactivity with the JC and BK viruses. The tension between sensitivity and specificity is especially important for this assay because BK and JC are ubiquitous in the human population and SV40 is apparently present only at low levels. Waning antibody titers pose challenges for current studies of people who might have been exposed to SV40 in the late 1950s through IPV or OPV. The challenges in applying current molecular biological techniques to the detection of SV40 DNA in tumor samples were discussed in the previous section.
The committee concludes that the biological evidence is moderate that SV40 exposure from the polio vaccine is related to SV40 infection in humans.
Summary of the Scientific Assessment
In summary, the committee’s scientific assessment concludes that moderate to strong lines of biological evidence support the theory that SV40 contamination of polio vaccine could contribute to human cancers. Specifically, the evidence is strong that:
-
SV40 contaminated some polio vaccine used from 1955–1963, and
-
SV40 has transforming properties in several experimental systems.
In addition, evidence has accumulated suggesting that SV4012 is likely present in some human tumors. The data regarding detection of SV40 in many but not all mesothelioma samples, coupled with the evidence for the oncogenic potential of SV40, suggest that SV40 could contribute to cancers in humans13. However, it is not clear
-
what proportion (if any) of the people exposed to the SV40-contaminated vaccine were infected,
-
what proportion (if any) of the human cancers in which SV40 is detected are caused by the SV40,
-
that the sole source of SV40 is due to the contaminated polio vaccine, or
-
that SV40-contaminated polio vaccine did or did not cause cancer in the vaccine recipients.
SIGNIFICANCE ASSESSMENT
The Immunization Safety Review Committee’s charge for the present series of studies includes consideration of the significance of the immunization safety issues for society—the context in which policy decisions must be made. Most of the issues reviewed by this committee concern vaccines presently in use. In the present case, however, current use of IPV is not in question. The issue instead is the possibility that the occurrence of certain cancers might be related to past use of SV40-contaminated polio vaccine between 1955 and 1963. Even today, this issue carries a major societal significance because exposure to the contaminated vaccine was so extensive and because cancer is such a serious and widely feared disease.
With poliomyelitis a serious health threat during the first half of the 20th century, IPV was eagerly welcomed in 1955. It was widely used in the United States and in many other countries before steps were taken to eliminate the inadvertent SV40 contamination. Household survey data indicated that by 1961, more than 98 million people in the United States had received one or more doses of IPV (Fraumeni et al., 1963). Shah and Nathanson (1976) estimated that 10 to 30 million of these people were exposed to SV40, but the numbers could easily be higher.
The evidence from animal studies has focused concern on four cancers: ependymoma, osteosarcoma, mesothelioma, and lymphoma. Non-Hodgkin’s lymphoma is the fifth most common cancer in the United States, and incidence rates have been rising over the past few decades. The other three cancers are serious but relatively rare, with 2,000 to 3,000 cases occurring each year. The incidence of ependymoma is highest in very young children, who also have the poorest prospects for long-term survival (Gurney et al., 1999a). Five-year survival rates for children 0 to 4 years of age are 46%, compared with 76% for 10- to 14-year-olds. Osteosarcomas are more likely to occur in adolescents. Survival has improved, but treatment can require amputation of an affected limb. In contrast, the incidence of mesothelioma is highest at older ages, and survival following diagnosis averages less than 2 years (Ho et al., 2001).
The committee’s review of the epidemiologic and biological evidence has shown that the effects of exposure to the contaminated polio vaccine remain uncertain, with important questions regarding the role of SV40 in human cancers unresolved. If future epidemiologic studies provided more compelling evidence for a causal link, the current evidence is sufficiently robust to suggest that the relative contribution of SV40 to overall risk would have to be small. Nevertheless, the possibility that millions of healthy individuals were exposed to a disease-causing agent could easily damage public confidence in the nation’s immunization program and the oversight groups responsible for assuring that the program is safe.
In previous reports (IOM, 2001a,b, 2002a,b), the committee has reviewed evidence that concerns about the safety of vaccines might reduce immunization rates and increase the levels of vaccine-preventable disease. In the 1970s, for example, concerns about the safety of pertussis vaccine were a factor in many countries in substantial declines in immunization rates that were followed by epidemics (Gangarosa et al., 1998). More recently, the claim that autism might be linked to measles vaccine or to the combination measles-mumps-rubella vaccine also appears to have contributed to lower immunization rates and higher levels of disease in the United Kingdom and Germany (Communicable Disease Report, 2001; Reuters, 2002). A recent survey in the United States found that almost a quarter of parents agreed that children received more immunizations than are good for them (Gellin et al., 2000).
The United States has a responsibility to thoroughly address health concerns stemming from the SV40 contamination of polio vaccine to ensure that any adverse health effects are identified, or to help produce the scientific evidence necessary for assurance that exposure to the contaminated vaccine has not had adverse effects.
The committee concludes that concerns about exposure to SV40 through inadvertent contamination of polio vaccines are significant because of the seriousness of cancers as the possible adverse health outcomes and because of the continuing need to ensure and protect public trust in the nation’s immunization program.
RECOMMENDATIONS FOR PUBLIC HEALTH RESPONSE
The scientific and policy issues considered by the committee lead to recommendations for targeted public health attention. The committee has found that the evidence is inadequate to determine whether recipients of the SV40-contaminated polio vaccine have an increased risk of cancer. However, because use of the polio vaccine was recommended by federal and national advisory bodies, because questions as to the possibility of continued transmission of SV40 in the population remain unresolved, and because understanding of the oncogenic potential of SV40 is at a pivotal point in the cycle of scientific discovery, public health attention in the form of policy analysis and further research is required.
Policy Review
Since 1961, FDA has required testing to ensure that polio vaccines used in the United States are free of SV40 contamination. The Advisory Committee on Immunization Practices, the American Academy of Pediatrics, and the American Academy of Family Physicians all now recommend exclusive use of IPV to immunize children and adults in the United States (CDC, 2000). The committee found no indication of any concern that SV40 contamination of IPV in the United States in use today might have escaped detection. Therefore, the committee does not recommend a policy review of polio vaccine by any of the national or federal vaccine advisory bodies on the basis of concerns about cancer risks that might be associated with exposure to SV40, because the vaccine in current use is free of SV40.
Claims have been made that some oral polio vaccines might have been contaminated after 1963 (Kops, 2000). The committee urges that FDA or other agencies address these claims to try to resolve the uncertainty regarding the possibility of exposure to SV40 after 1963. Appropriate assumptions about exposure are essential for conducting valid epidemiologic analyses of the risks that might be associated with contaminated OPV.
Policy Analysis and Communication
The ability of researchers to use epidemiologic studies to assess the possible association of SV40 with certain cancers has been hindered in part by the small numbers of cases for most of the cancers of concern, but also by substantial uncertainty in classifying individuals as exposed or unexposed.
The uncertainty as to exposure arises in large part because of lack of detailed information on the levels of SV40 in the vaccine and on who received the vaccine. Testing of stored samples of IPV used in 1955 showed that the levels of SV40 varied among vaccine lots and that some lots were not contaminated (Fraumeni et al., 1963). But samples of vaccine produced through the remainder of the period of likely contamination (1955–1961) were not available for testing, leaving investigators with little basis for identifying differences in SV40 exposure among the vaccinated population. Misclassification of exposure would result in a bias toward the null hypothesis of no difference between the “exposed” and “unexposed” groups.
The committee hopes that contamination of a vaccine never occurs again, but also considers it prudent to have a comprehensive plan in place for prevention of contamination, as well as for response and communication should such an event occur. Pieces of such a plan already exist within the various agencies with responsibility for assuring the safety of vaccines. For example, FDA has regulatory authority over the production of vaccines. Currently, all vaccines licensed by the FDA are required to fulfill general safety, sterility, and purity requirements (Code of Federal Regulations, 2001). For example, the cell substrates used to produced the MMR vaccine are from flocks free of the avian leukosis virus. The MMR vaccine also undergoes testing for adventitious viral activity. Cell lines used to produce the poliovirus vaccine are tested for (in addition to SV40) tuberculosis, herpes viruses, measles, and other infectious agents (CDC, 1997). However, the committee is not aware of a comprehensive system that is transparent and clearly understandable to, and accessible by, the public. The most recent comprehensive plan put together by the federal government on vaccine safety does not address contamination issues (NIH, 1998).
The committee recommends that the appropriate federal agencies develop a Vaccine Contamination Prevention and Response Plan. The appropriate agencies should be given the authority and resources to implement the plan once it is in place. This plan should identify the procedures already in place or those that need to be developed, for example, to prevent contamination of vaccine during the manufacturing process. In addition, the plan should include strategies for routine assessment of vaccine for possible contamination; notification of public health officials, health care providers, and the public if contamination occurs; identification of recipients of contaminated vaccine; and surveillance and research to assess health outcomes associated with the contamination. Clearly the plan will need to allow for the scientific and technical uncertainties surrounding an assertion that contamination has occurred or is possible. Implementation of the plan will require considerable judgment as to the level of response required to deal with a specific contamination concern. Because the plan will involve multiple agencies and offices, the National Vaccine Program Office is probably the best positioned to organize and coordinate the development of the plan.
The development of the plan should be the subject of broad and open discussion by the regulators, policymakers, manufacturers, researchers, vaccine administrators, and the public. Once a plan is developed, a communication campaign should be undertaken to inform the public and medical practitioners. Open discussion and communication are important to ensure that trust in the vaccine supply is deserved and is widespread. The committee noted several specific issues that should be considered in developing a Vaccine Contamination Prevention and Response Plan.
A program to store samples from each vaccine lot approved for release should be considered. This would make it possible to test for contaminants should safety questions arise well after the vaccine was used or as new detection techniques become available. Manufacturers are currently required to store a sample of each released lot only until the expiration date of that lot.
Consideration should also be given to developing better mechanisms to identify the recipients of vaccine from specific lots. The committee recognizes that this is increasingly difficult. With a more mobile population and less continuity of medical care, an individual’s immunization records are often scattered or incomplete. Vaccinations provided at public clinics or other sites that are not connected with usual sources of medical care may not be recorded. Moreover, regulations intended to protect individual privacy restrict the use of medical records. The large cohorts that were included in the some of the epidemiologic studies reviewed here (e.g., Heinonen et al., 1973; Fraumeni et al., 1970; Innis, 1968; Stewart and Hewitt, 1965) would be almost impossible to establish without laying the appropriate groundwork (e.g., consent for contact for future research studies) at the time of vaccination.
The Vaccine Safety Datalink (VSD) program has been one attempt to improve access to data linking health outcomes with specific vaccine exposures. This collaboration between CDC and several health maintenance organizations covers about 2.5% of the U.S. population (CDC, 2001). It has been used to investigate certain questions, including intusseption following rotavirus vaccine, and the risk of seizures following vaccination, but larger populations are needed to study rare outcomes, like most of the cancers of concern in this report.
Immunization registries may be another way to meet the need for information on vaccine recipients, but they are still evolving. Most are currently designed to capture information on immunizations given to children under 6 years of age. However, registries are not yet available throughout the country and where they are operating, some immunization providers do not submit data (CDC, 2002b). Even with the benefit of registry data, the challenges of tracing individuals remain. And in their current form, immunization registries do not capture information on vaccine given to adolescents or adults.
Research
The committee notes that data are accumulating rapidly on many fronts on the potential for viruses to cause cancers in humans, particularly if exposure is early in life. The committee supports continued research in viral oncogenesis broadly, independent of the question of SV40-contamination of polio vaccine. However, the research recommendations are focused specifically on the question of the polio vaccine, in keeping with the charge to the committee.
The committee recommends development of sensitive and specific serologic tests for SV40. These would be helpful to resolve the question as to whether or not the SV40 exposure led to infection.
The committee recommends the development and use of sensitive and specific standardized techniques for SV40 detection. Included in these efforts should be a documentation that: 1) all test specimens are masked, 2) positive and negative control tissues are both used and are both subjected to the same processing procedures as test specimens, 3) samples are tested in replicate, and 4) an adequate sample of tissue is available.
The committee recommends that once there is agreement in the scientific community as to the best detection methods and protocols, pre-1955 samples of human tissues should be assayed for the presence or absence of SV40 in rigorous, multicenter studies. These tests would not address the question of whether or not SV40 can cause cancer, but they could influence the interpretation of some epidemiologic and clinical analyses. They also would be relevant for discussion of the relative contribution of contaminated polio vaccine to the burden of SV40 infection in humans.
The committee recommends further study of the transmissibility of SV40 in humans. This will help confirm whether and why SV40 or antibodies specific for SV40 are detected in individuals who have no known exposure to potentially contaminated polio vaccine, animals or laboratory contact. Resolving the issue of transmissibility of SV40 is not directly relevant for a causality assessment, but it would be useful in some of the ancillary debates about the role of the polio vaccine contamination in the cancer burden.
In addition to the research recommended above, it is important to resolve the extent of SV40 contamination of past polio vaccine. The uncertainty of exposure makes interpretation of the epidemiologic studies very problematic. In addition, concerns that the oral polio vaccine had been more widely contaminated than assumed require attention. The issue should be resolved publicly to the satisfaction of the public as well as the scientific and policy-making communities.
If researchers can pursue these strategies and obtain a better understanding of SV40 exposure and methods of detection, more meaningful case-control studies can be undertaken to help resolve the question of causality. Until some of the technical issues are resolved, the committee does not recommend additional epidemiologic studies of people potentially exposed to the contaminated polio vaccine.
SUMMARY
Some of the polio vaccine administered from 1955–1963 was contaminated with simian virus 40 (SV40). The virus came from the monkey kidney cell cultures used to produce the vaccine. Most, but not all, of the contamination was in the inactivated polio vaccine (IPV). Once the contamination was recognized, steps were taken to eliminate it from future vaccines. There have been many questions as to the effects on people who received the contaminated vaccine. SV40 has biological properties consistent with a cancer-causing virus, but researchers have not conclusively established whether or not it could cause cancer in humans. Studies of groups of people who received polio vaccine during 1955–1963 provide evidence of no increased cancer risk.
However, because these epidemiologic studies are sufficiently flawed, the Institute of Medicine’s Immunization Safety Review Committee concluded that the evidence was inadequate to conclude whether or not the contaminated polio vaccine caused cancer. In light of the biological evidence supporting the theory that SV40-contamination of polio vaccines could contribute to human cancers, the committee recommends continued public health attention in the form of policy analysis, communication, and targeted biological research. Box 3 summarizes the committee’s conclusions and recommendations.

BOX 3
Committee Conclusions and Recommendations. SCIENTIFIC ASSESSMENT Causality Conclusions
Footnotes
- 1
-
For a discussion of the evolution of the terminology concerning biological mechanisms, see the committee’s earlier reports (IOM, 2001a,b, 2002a,b).
- 2
-
IPV was licensed and widely distributed in 1955, however exposure to SV40 may have also occurred in the 1954 field trial of IPV.
- 3
-
Current formulations of IPV and OPV available in the United States are required by the FDA to be free of SV40. The IPV produced today uses poliovirus grown on Vero cells, a continuous line of green monkey kidney cells. OPV is no longer produced in the United States, but as the recommended vaccine to control polio outbreaks, a stockpile of OPV is available for these purposes (CDC, 2000). The OPV was produced in the United States in monkeys raised in colonies free from SV40 or grown in Vero cells and was screened for viruses, including SV40 (Sutter et al., 1999).
- 4
-
During the same period, SV40 also contaminated an experimental respiratory syncytial virus vaccine given to about 100 adults and a licensed adenovirus vaccine given to about 100,000 military inductees (Shah and Nathanson, 1976).
- 5
-
Incidence rates are age-adjusted to the 2000 U.S. standard population.
- 6
-
Incidence rates are age-adjusted to the 1970 U.S. standard population.
- 7
-
As osteosarcoma is predominantly classified as a childhood cancer, incidence rates are most often reported for persons under 20 years of age.
- 8
-
Incidence rates are age-adjusted to the 1970 U.S. standard population.
- 9
-
Another type of lymphatic cancer, Hodgkin’s Disease (HD), is distinguished from NHL by the presence of Reed-Sternberg cells.
- 10
-
NHL incidence rates are age-adjusted to the 2000 U.S. standard population.
- 11
-
Asbestos is not the topic of the committee’s report and those data will not be discussed.
- 12
-
In the form of virus, viral fragments, DNA, or SV40 gene products
- 13
-
The data regarding mesothelioma are more substantial and more abundant than for other cancers, such as NHL, osteosarcoma, or ependymomas.
National Center for Biotechnology Information, U.S. National Library of Medicine 8600 Rockville Pike, Bethesda MD, 20894 USA